Ever wondered how molecules behave in different environments? You might be surprised to learn that the speed of molecules can vary significantly depending on their surroundings. Imagine a crowded room where everyone’s bumping into each other versus an open field where you can run freely. This simple analogy can help you grasp how molecules move in a vacuum compared to other states.
Key Takeaways
- Molecular Motion Dynamics: Molecules exhibit varying speeds based on their environment, with motion influenced by temperature, pressure, phase of matter, molecular mass, and interactions with other molecules.
- Vacuum Characteristics: A vacuum, characterized by low particle density and reduced pressure, allows molecules to move more freely and rapidly due to minimal collisions.
- Increased Speed in Vacuum: Molecules typically move faster in a vacuum because the absence of other particles eliminates obstacles, resulting in greater kinetic energy and motion.
- Experimental Support: Experiments, such as molecular beam studies, demonstrate that molecules in a vacuum travel faster than those in atmospheric conditions, confirming the unique speed characteristics of vacuum environments.
- Real-World Applications: Understanding molecular behavior in a vacuum is crucial across various fields, including astrophysics, materials science, and pharmaceuticals, affecting processes like chemical reactions and material deposition techniques.
Understanding Molecular Motion
Molecular motion refers to the movement of molecules, which plays a vital role in understanding how different environments impact their speed. In a vacuum, the behavior of molecules changes compared to those in other mediums, such as liquids or gases.
Definition of Molecular Motion
Molecular motion describes the movement patterns of molecules due to energy they possess. This energy results from temperature changes, pressure variations, or environmental conditions. It’s essential to recognize that molecules range from vibrating slowly to colliding energetically, depending on factors like their surroundings or phase of matter.
Factors Influencing Molecular Speed
Several factors determine the speed at which molecules move:
- Temperature: Higher temperatures increase kinetic energy and result in faster-moving molecules. For example, water molecules in boiling water move much faster than those in ice.
- Pressure: Increased pressure can lead to closer proximity among molecules, influencing their interactions and speed. Gases under high pressure display different behaviors compared to those at a lower pressure.
- Phase of Matter: Molecules behave differently in solids, liquids, and gases. Gases move freely and rapidly, while solids have limited movement.
- Molecular Mass: Lighter molecules tend to move faster than heavier ones. For instance, hydrogen gas molecules travel faster than oxygen gas molecules at the same temperature.
- Presence of Other Molecules: Interactions with other molecules can either hinder or facilitate movement. In a vacuum, there are no other molecules to collide with, allowing for unobstructed motion.
The Concept of Vacuum
Understanding a vacuum is essential for grasping how molecules behave. A vacuum represents an environment where matter is almost entirely absent, leading to unique molecular characteristics.
What Is a Vacuum?
A vacuum is defined as a space with significantly reduced pressure, resulting in very few particles. It can exist naturally, as in outer space, or be created artificially, such as in a vacuum chamber. The absence of air or other gases in a vacuum allows for unhindered molecular motion, which differs from molecular behavior in more crowded environments.
- Low Particle Density: In a vacuum, the number of molecules is minimal. This sparse distribution allows individual molecules to move freely without frequent collisions.
- Reduced Pressure: A vacuum exhibits lower pressure compared to atmospheric conditions. This factor influences molecular speed, as there’s less force acting against molecular motion.
- Temperature Influence: Although temperature affects molecular speed in all environments, in a vacuum, temperature becomes a critical factor. Higher temperatures contribute to increased energy, thus higher speeds.
- Isolation from External Forces: A vacuum protects molecules from external influences, such as air resistance. The absence of these forces leads to pure kinetic motion based only on molecular energy.
- Behavioral Differences: Molecules in a vacuum experience fewer interactions, allowing them to move more freely than in liquids or gases where collisions are common. This behavior illustrates significant variations in molecular dynamics across different environments.
These characteristics explain why understanding vacuum conditions is essential for comprehending molecular motion and speed.
The Relationship Between Molecules and Vacuum
A vacuum creates a unique environment for molecular behavior. In this space, particle density decreases significantly, affecting how molecules move.
Do Molecules Move Faster in a Vacuum?
Yes, molecules generally move faster in a vacuum. In this setting, there are fewer particles to collide with, allowing for freer motion. Without obstacles, kinetic energy increases, leading to higher speeds. For instance, in a vacuum, gas molecules can travel long distances without interacting with other molecules, differing from their behavior in air or liquids where they frequently collide.
Experimental Evidence Supporting Molecular Speed in a Vacuum
Numerous experiments confirm that molecular speed increases in a vacuum. One example involves molecular beam experiments, where beams of gas molecules are directed into a vacuum chamber. Results show that these molecules travel much faster than those in atmospheric conditions. Researchers also use vacuum systems in various scientific studies to measure molecular velocities accurately. These findings indicate that under vacuum conditions, molecules demonstrate distinct speed characteristics compared to environments filled with other particles.
Real-World Implications
Understanding how molecules behave in a vacuum has significant implications across various domains, from experimental science to industrial applications.
Applications in Science and Technology
Molecular behavior in a vacuum plays a critical role in fields like astrophysics and materials science. For instance, in the study of space, scientists analyze how molecules behave in the vacuum of outer space to understand planetary atmospheres, solar winds, and cosmic phenomena. In semiconductor manufacturing, vacuum conditions facilitate the deposition of thin films, allowing precise control over material properties. Innovations in vacuum technology enable more efficient processes, improving the performance of products ranging from electronics to pharmaceuticals.
Effects on Chemical Reactions
Chemical reactions often depend on molecular collisions. In a vacuum, fewer molecules lead to reduced collision frequency, which alters reaction rates. This environment allows for unique experiments, such as those in mass spectrometry, where molecules interact minimally with their surroundings, improving detection accuracy. Reactions that are slow or require specific conditions at atmospheric pressure may proceed at different rates in a vacuum. Understanding these dynamics enables researchers to optimize reaction conditions, leading to improved yield and efficiency in chemical production.
Conclusion
Understanding how molecules behave in a vacuum opens up fascinating possibilities. With fewer collisions and increased kinetic energy, these tiny particles can move more freely and quickly. This knowledge not only enhances your grasp of molecular motion but also has practical applications in various fields.
Whether you’re exploring the depths of space or working on the latest technology in materials science, the implications of molecular speed in a vacuum are significant. Embracing these concepts can deepen your appreciation for the intricate dance of molecules and their impact on our world. Keep this in mind as you delve further into the exciting realm of molecular science.
Frequently Asked Questions
What is molecular motion?
Molecular motion refers to the movement of molecules in different states of matter. Factors such as temperature, pressure, and molecular mass affect how quickly and actively molecules move. Understanding these principles is fundamental to various scientific fields.
How do temperature and pressure affect molecular movement?
Higher temperatures typically increase molecular speed due to greater kinetic energy, while higher pressure can compress molecules, affecting their spacing and collision rates. Both factors play a crucial role in determining molecular behavior in different environments.
What is a vacuum?
A vacuum is a space devoid of matter, characterized by low particle density and reduced pressure. This unique environment enables molecules to move with fewer collisions, resulting in increased speed and kinetic energy compared to conditions with atmospheric pressure.
How does molecular behavior differ in a vacuum?
In a vacuum, molecules experience fewer collisions due to low particle density, allowing them to travel longer distances and move faster. This behavior contrasts with atmospheric conditions, where increased interactions slow down molecular motion.
What experimental evidence supports faster molecular movement in a vacuum?
Molecular beam experiments provide evidence that molecules indeed move faster in a vacuum. These experiments demonstrate distinct speed characteristics in various environments, confirming the influence of reduced particle density on molecular behavior.
Why is understanding molecular motion in a vacuum important?
Understanding molecular motion in a vacuum has real-world implications in fields like astrophysics, materials science, and semiconductor manufacturing. It helps researchers comprehend how vacuum conditions affect chemical reactions, enhancing detection accuracy and optimizing experimental setups.
How does a vacuum influence chemical reactions?
A vacuum reduces collision frequency among molecules, which may alter reaction rates. This unique environment can lead to new experimental techniques, such as mass spectrometry, improving detection accuracy and enabling optimized reaction conditions.
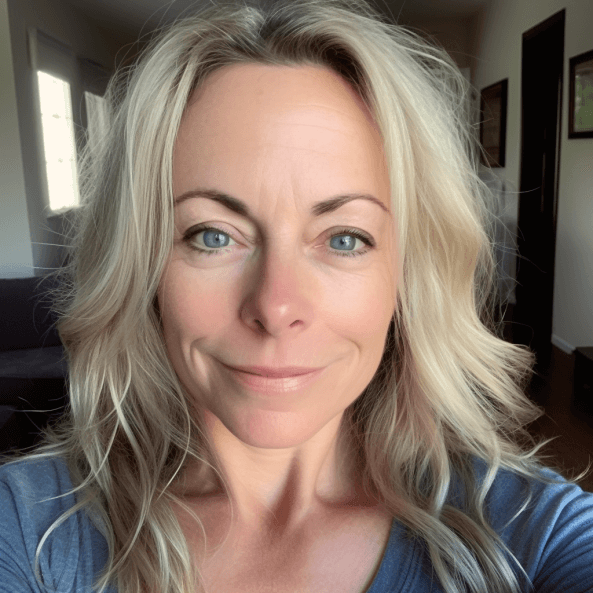