Have you ever wondered how sound travels through different environments? Imagine you’re floating in space, surrounded by silence. It raises an interesting question: can compressional waves, like sound waves, move through the vacuum of space?
Key Takeaways
- Compressional Waves Require a Medium: These waves, including sound waves, cannot travel through a vacuum as they need particles to transmit energy.
- Speed Variation by Medium: Compressional waves travel fastest in solids due to tightly packed particles, slower in liquids, and slowest in gases.
- Impact of Vacuum on Sound: In space, the absence of a medium means sound waves can’t propagate, leading to silence where auditory communication fails.
- Scientific Evidence Reinforces Theory: Experimental studies confirm that compressional waves do not travel in a vacuum, validating theoretical understanding.
- Communication Challenges in Space: Traditional sound-based communication is impossible in space, necessitating the use of electromagnetic waves for effective communication.
- Redundancy and Signal Boosting: Ensuring multiple communication systems and using technology like signal boosters are vital for maintaining reliable communication in space conditions.
Understanding Compressional Waves
Compressional waves, also known as longitudinal waves, are a type of mechanical wave that requires a medium to travel. These waves consist of alternating compressions and rarefactions, where particles of the medium move parallel to the direction of the wave energy.
Definition and Characteristics
Compressional waves occur when a disturbance causes particles in a medium to vibrate back and forth along the same line as the wave travels. This movement creates areas of high pressure (compressions) and low pressure (rarefactions). Key characteristics include:
- Medium Requirement: Compressional waves require a solid, liquid, or gas. They can’t travel through a vacuum.
- Speed Variation: The speed of compressional waves depends on the density and elasticity of the medium. For example, they travel faster in solids than in liquids or gases.
- Energy Transfer: These waves transmit energy through the medium without permanently displacing it.
Types of Compressional Waves
Different types of compressional waves exist, each with specific applications and characteristics. Common types include:
- Sound Waves: These waves travel through air or other mediums, where they are detected by our ears.
- P-Waves: A type of seismic wave generated by earthquakes. P-waves are the fastest seismic waves and can move through both solid and liquid layers of the Earth.
- Water Waves: These waves in bodies of water exhibit both longitudinal and transverse characteristics, affecting the water’s surface.
Understanding these types helps illustrate how compressional waves function in various environments, reinforcing their dependence on a medium for transmission.
The Vacuum of Space
The vacuum of space lacks matter, making it an intriguing environment. Understanding how compressional waves interact—or fail to interact—with this vast emptiness clarifies their fundamental properties.
Definition and Properties
Compressional waves, or longitudinal waves, are characterized by the movement of particles in the same direction as the wave. This motion creates alternating regions of compression and rarefaction. They require a medium—like air, water, or solid substances—to travel. In a vacuum, where no particles exist, these waves cannot propagate.
This inability connects primarily to the following properties:
- Medium Requirement: Compressional waves need particles to transmit energy. Without a medium, waves cease to exist.
- Speed Variation: The speed of compressional waves is influenced by the medium’s density and elasticity. Sound waves, for example, travel faster in water than in air due to water’s higher density.
Absence of Medium for Sound
In space, sound waves cannot travel because of the absence of air or any other medium. For example, astronauts outside a spacecraft cannot hear each other due to the vacuum. Sound requires particles for vibration and transmission; void of those, it can’t exist.
Understanding this principle underscores why compressional waves fail to traverse the vacuum of space. Knowing that sound—which is a type of compressional wave—relies on air, you grasp why communication in space needs technological methods, not sound.
Behavior of Compressional Waves in Different Mediums
Compressional waves exhibit varied behaviors depending on the medium through which they travel. Understanding these behaviors clarifies why compressional waves cannot traverse the vacuum of space.
Solids
Compressional waves travel fastest in solids due to closely packed particles. When you strike a solid object, like a metal rod, the vibration quickly propagates through the material as particles compress and expand. For example, seismic P-waves, which are compressional waves, move through the Earth’s crust and are detected by seismographs. The high density and rigidity of solids allow these waves to maintain their energy efficiently, leading to rapid transfer.
Liquids
Compressional waves also travel through liquids, though not as quickly as in solids. In liquids, particles are less tightly packed and can move more freely. An excellent example is when you drop a stone into water; the resulting ripples demonstrate how compressional waves propagate through the liquid medium. The speed of these waves depends on the liquid’s density and temperature, with warmer liquids generally allowing faster wave transmission.
Gases
Compressional waves travel slowest in gases because of the large distances between particles. For instance, sound waves are a familiar example of compressional waves in air. When someone speaks, the vocal cords create compressions and rarefactions in the surrounding air molecules, allowing sound to travel. The lower density and elasticity of gases compared to solids and liquids result in slower wave speeds. Temperature and pressure changes in the gas can also impact how quickly these waves move.
Scientific Perspectives on Compressional Waves in Space
Understanding compressional waves reveals important insights into their behavior in different contexts. These waves can only propagate through a medium, which fundamentally limits their place in the vastness of space.
Theoretical Framework
Compressional waves, or longitudinal waves, require a medium like solid, liquid, or gas to transport energy. In theory, for waves to exist, particles must be able to bounce against one another. Since space is a vacuum, it lacks these particles. Thus, compressional waves cannot travel through the emptiness of space. This principle supports the idea that sound waves, for example, lose their ability to transmit in a vacuum, reinforcing the idea that a medium is crucial for wave propagation.
Experimental Evidence
Experimental studies consistently confirm the behavior of compressional waves in various mediums. For instance, sound waves travel at approximately 343 meters per second in air, while seismic P-waves can exceed 5,000 meters per second in dense rock. In vacuum conditions, like those simulated in a laboratory, sound waves do not propagate at all, validating the theoretical framework. Experiments using vacuum chambers demonstrate that when an audio source is activated inside a vacuum, no sound reaches outside, illustrating the absence of wave transmission due to lack of particles. This scientific evidence provides clarity on the nature of compressional waves and their interaction with different conditions, particularly the challenges faced in the vacuum of space.
Implications for Communication in Space
Understanding compressional waves shapes the framework for communication in space. Given their requirement for a medium, the lack of a medium in the vacuum of space presents significant challenges.
Challenges Faced
- Transmission Limitations: Compressional waves can’t travel through a vacuum, making traditional sound-based communication impossible. Communication systems relying on sound transmission aren’t applicable in space.
- Delayed Signals: Electromagnetic waves, like radio waves, travel through space but can encounter delays due to cosmic phenomena. This delay affects real-time communication between spacecraft and Earth.
- Equipment Constraints: Space missions often limit the technology available for communication. Developing equipment with reliable functionality in varying conditions adds complexity.
- Use of Electromagnetic Waves: Switching to electromagnetic waves for communication addresses the vacuum issue. Devices like radios operate on this principle, allowing effective communication in space.
- Signal Boosting Technology: Implementing signal boosters or relays can enhance communication efficiency. Deploying satellites in orbit assists with transmitting signals over vast distances.
- Redundant Systems: Developing multiple communication pathways ensures backup options are available. Incorporating diverse technologies, like laser communication, can create redundancy.
- Time Encoding Techniques: Leveraging time-stamped signals can make it easier to manage communication delays. This approach can help synchronize information shared across long distances.
Conclusion
Compressional waves are fascinating but they can’t travel through the vacuum of space. Without a medium like air or water they simply can’t propagate. This limitation has significant implications for communication in space where traditional sound waves fall flat.
As you explore the cosmos or think about future space missions it’s essential to remember the role of different wave types. While compressional waves may not be your go-to for interstellar communication you can rely on electromagnetic waves to bridge that gap. Embracing technology and innovative solutions will help you navigate the challenges of space communication effectively.
Frequently Asked Questions
What are compressional waves?
Compressional waves, or longitudinal waves, are waves that require a medium to travel through. Unlike transverse waves, where particles move perpendicular to the wave direction, in compressional waves, particles move back and forth in the same direction as the wave.
How do compressional waves propagate?
These waves propagate by creating compressions and rarefactions in the medium as particles vibrate. The wave moves through the medium by transferring energy from one particle to another, allowing the wave to travel.
What affects the speed of compressional waves?
The speed of compressional waves depends on the density and elasticity of the medium. Generally, they travel fastest in solids, more slowly in liquids, and slowest in gases due to the arrangement and proximity of the particles.
Why can’t compressional waves travel through space?
Compressional waves cannot travel through space because they require a medium—such as a solid, liquid, or gas—to propagate. In the vacuum of space, where there are no particles, these waves have no medium to move through.
How do compressional waves relate to sound waves?
Sound waves are a type of compressional wave that travels through air, liquids, or solids. They exhibit the same principles of propagation, creating areas of compression and rarefaction as sound energy travels through the medium.
What are P-waves?
P-waves, or primary waves, are a type of compressional wave that occurs during seismic events. They are the fastest seismic waves and can travel through solids, liquids, and gases, making them crucial for understanding earthquakes.
How do temperature and medium density affect wave speed?
Higher temperature typically increases the speed of compressional waves as particles move faster and transmit energy more quickly. Similarly, a denser medium allows for quicker wave propagation due to the closer arrangement of particles.
What are the implications for communication in space?
In space, the inability of compressional waves to travel poses challenges for communication. Without a medium, signal transmission is limited, leading to delayed signals and equipment constraints, which require alternate methods, such as using electromagnetic waves.
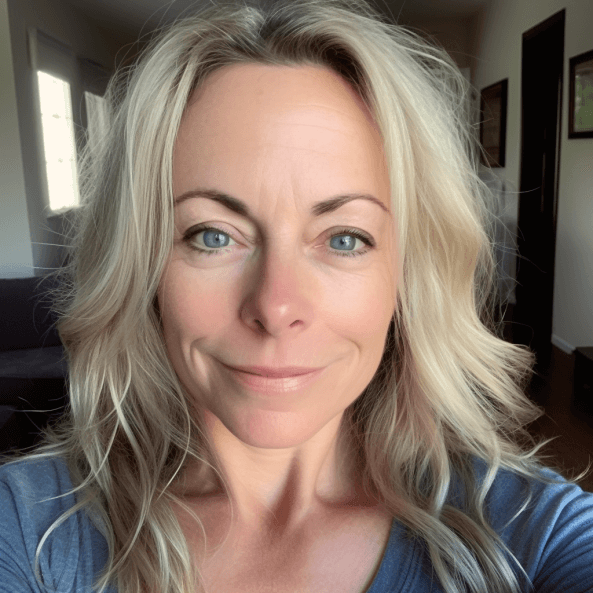